How Economics Shapes Science
Stephan reveals how economic incentives have made American science risk averse and turned our universities into lobbying superpowers and employment pyramid schemes.
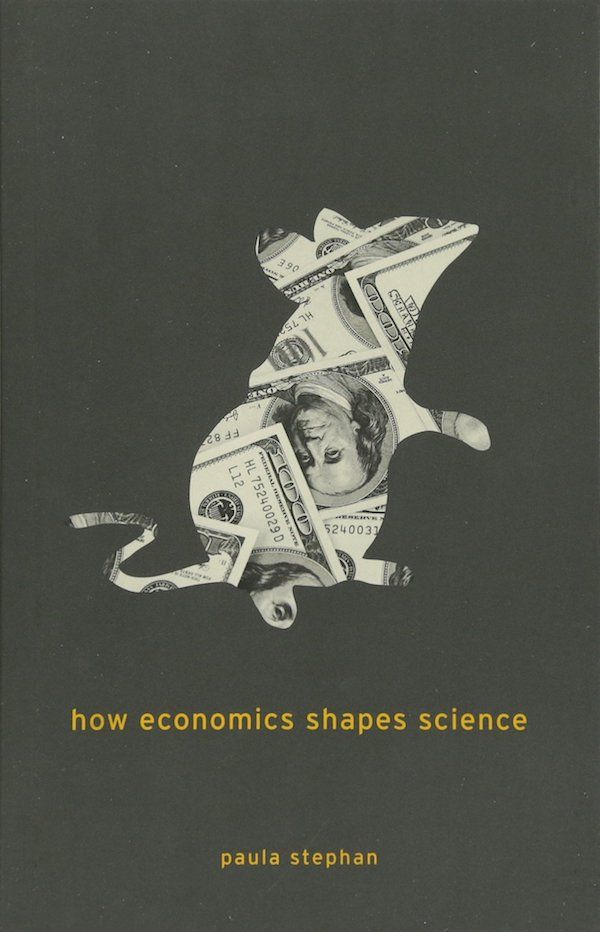
My relentless cynicism drives me to always "follow the money." So for my 2017 theme on "The Integrity of Western Science", my dark, jaded little heart leapt with joy when I discovered "How Economics Shapes Science". Stephan hands us a heavy, dry bucket of facts - but the story that emerges is central to understanding the current dysfunctions of American science. By tracing the evolution of funding streams for science, Stephan reveals how the resulting incentives have made American science risk averse and turned our universities into lobbying superpowers and employment pyramid schemes.
Universities and medical schools receive over $55 billion a year for research (that's over $500 per full time employee in the US). Some powerhouse universities, like Stanford, get over 20% of their revenue from research funding (over $750 million a year). Because almost all of this money comes from the government, many universities now have full-time lobbyists in DC to advocate for more research funding. The cynic in me notes that the university administration takes an enormous chunk as "overhead" (Yale, for example, takes up 70%!!!) and this is a contributor to the explosion of administrative headcount in the modern university. But simultaneously, the universities have reduced their funding of research, shifting risk off of the university and on to the individual investigator. This means that researchers are more dependent than ever on government grants to sustain their research (and even to pay their salaries). Indeed, some medical school professors now get tenure "packages" that include no salary payments at all from the university! Because granting agencies like to give relatively short-term grants and to see visible progress, researchers often choose to pursue incremental, less-risky research to ensure that they don't end up without funding. Furthermore, the grant process rewards past performance in a way that makes it difficult for new researchers to establish a foothold of their own. Although Stephan paints a grim picture, she also points out rays of hope like the Howard Hughes Medical Investigators program that are addressing some of these challenges at a small scale.
As the husband of a current Ph.D. student, I was particularly interested in what Stephan had to say about the employment dynamics for post-grads and new professors. Unfortunately, she doesn't have great news:
In certain ways, the research enterprise itself at U.S. universities resembles a pyramid scheme. In order to staff their labs, faculty recruit PhD students into their graduate programs with funding and the implicit assurance of interesting research careers... the number of tenure-track positions has failed to keep pace with the large number of newly minted PhDs. It is not uncommon for recent graduates to feel that the system has not delivered what it promised.
This extreme competition for tenure-track positions is another factor that contributes to the risk-aversion of modern academic scientists. It also drives the salaries of post-docs down and results in a surprising dynamic. White men are abandoning academic career paths for more secure and profitable employment in industry. Their spots are being filled by women and by foreign grad students (especially from China) for whom these "low" salaries are quite reasonable compared to what is available in their home countries. Surveying the research dynamics in Europe and Asia, Stephan does touch briefly on the world of research in China, noting that:
"To obtain major grants in China, it is an open secret that doing good research is not as important as schmoozing with powerful bureaucrats and their favorite experts."
Of course, this corruption isn't limited to China. Old Moldbug has plenty to say about the government-academia "Cathedral" complex and Stephan has a brief section on fraud and undisclosed funding sources for biomedical research (usually by pharma). But Stephan shrugs off outright fraud as a pretty minimal part of the modern research system. Instead, the major axe she grinds in this book is risk aversion and how it slows down scientific progress:
The current university research system in the US also discourages research that could disprove theories. To quote an official with a disease foundation, who asked not to be identified, "The way science careers are structured, big labs get established based on a theory or a target or a mechanism, and the last thing they want to do is disprove it and give up what they're working on. That's why we have so many targets in studying this disease. We'd like people to work on moving them from a 'maybe' to a 'no,' but it's bad for careers to rule things out: that kind of study tends not to get published, so doing that doesn't advance people's careers.
Stephan's book gave me a clear framework for thinking about how money influences modern research. But it's dry as a bone and likely only of interest to specialists. If you are looking to learn more about some of the problems with modern science, "Rigor Mortis" covers similar topics for a popular audience.
My highlights below
Chapter One - What Does Economics Have To Do with Science?
In the United States, for example, approximately 75 percent of all articles published in scientific journals are written by scientists and engineers working at universities and medical schools. Of equal importance, almost 60 percent of basic research is conducted at universities and medical schools.
The personnel costs of a typical university lab with eight researchers is about $350,000 after fringe benefits but before taking into account the cost of the principal investigator's time or indirect costs.
The United States spends between 0.3 and 0.4 percent of its gross domestic product (GDP) on research and development at universities and medical schools. This represented almost $55 billion dollars in 2009 or approximately $170 per person.
Universities respond to incentives. In the early 2000s, universities went on an unprecedented building spree, developing new research facilities in the biomedical sciences. Within less than five years, construction and renovation costs for biomedical research facilities accelerated from $348 million annually to $1.1 billion annually at U.S. medical schools. (All figures are in 1990-adjusted dollars.) The reason: the budget for the National Institutes of Health, the major funder of research in the biomedical sciences, doubled between 1998 and 2003, opening a panoply of what universities perceived to be new opportunities to expand their research efforts and, in the process, enhance their reputation. It was not the first time that U.S. medical schools responded to financial incentives. The substantail expansion of medical colleges over the past 40 years is widely attributed to the adoption of Medicare and Medicaid in 1965, which provided university medical schools with a new source of revenue.
The number of articles submitted to the journal Science, for example, is significantly related to whether the scientist's home country offers a bonus or other monetary reward for publishing in the journal.
Although rare, events such as these occur with sufficient frequency that, on the campus of almost every research university in the United States, two or three faculty members have become wealthy as a result of their research.
Full professors at the top of their game employed at private research universities in math earned an annualized salary of $180,000 a year in 2006 in the United States. Comparably ranked full professors at public universities earned $150,000. Those in the biological sciences earned $277,700 at private research universities; those at public universities earned $200,000.
A common way to measure the reputation of a scientist is to count the number of citations to an article or to the entire body of the scientist's work. The h-index, a citation-based method for measuring the impact of a scientist's work, has gained considerable use in recent years.
A reward structure that encourages scientists to share their discoveries in a timely manner is highly functional. The reason: knowledge has characteristics of what economists call a public good. It is nonexcludable and nonrivalrous.
The lack of monetary incentives could lead to what economists refer to as "market failure," with society producing considerably less research than is socially desirable."Society, however, is more ingenious than the The priority system has evolved in science to create a reward system that encourages the production and sharing of knowledge. The very act of staking a claim requires scientists to share their discoveries with others.
It not only takes time; considerable investment and know-how are required to translate research into new products and processes. Industry, not academe, excels in doing this. In singing the praises of academic research, one should not forget that innovations come from research and development-and development has long been the domain of industry.
Relationships between universities and industry are fed by the constant supply of new talent that universities send to industry.
faculty receive about 6 percent of their research funds from industry
First, beware of attributing causality from correlation. Second, if at all possible, think of the counterfactual. Without a counterfactual, it is not possible to assess the impact of a policy on outcomes. The fact, for example, that the research that led to the MRI and the atomic clock originated in academic settings does not prove that the two would not have been invented elsewhere.
As used in this book, basic research refers to research directed at furthering fundamental understanding; applied research is directed at solving practical problems. Increasingly, and particularly in certain fields, such as the biomedical sciences, the distinction is somewhat moot. Researchers can have the dual goal of advancing fundamental understanding as well as solving practical problems.
Productivity in science is highly skewed: approximately 6 percent of scientists and engineers write 50 percent of all published articles.
But peer review has its downside. The large amount of time required to apply for and administer grants diverts scientists from spending time doing research. The peer-review system also discourages risk taking. Failure is not rewarded.
Pyramid schemes are not limited to Wall Street or to salesmen - they exist in science, especially in the biomedical sciences, where faculty persist in recruiting graduate students and postdocs to work in their labs despite strong evidence that a sufficient number of research jobs for those in training do not exist.
We also see evidence that increased numbers of foreign born depress salaries, especially salaries of postdocs, and thereby may discourage U.S. citizens from choosing careers in science and engineering.
Chapter Two - Puzzles and Priority
The philosopher of science David Hull describes scientists as innately curious and suggests that science is "play behavior carried to adulthood."
"The pursuit of reputation in the eyes of others," according to philosopher and psychologist Rom Harre, "is the overriding preoccupation of human life.""... "Give me enough ribbon,"Napoleon reportedly said, "and I can conquer the world.""
Reputation is built in science by being the first to communicate a finding - by establishing what the sociologist of science Robert Merton refers to as the priority of discovery.
RSA, an algorithm for a public-key cryptosystem and the algorithm of choice for encrypting Internet credit-card transactions, was published in 1977 by Ron Rivest, Adi Shamir, and Leonard Adleman (hence the name RSA). But Clifford Cocks, a mathematician working for the British intelligence agency GCHQ, described an equivalent methodology in a 1973 document that, due to its top-secret classification, was not revealed until 1997.
Science, a leading if not the leading multidisciplinary journal in science, has the explicit policy of asking referees to return their reviews within seven days of receipt of the manuscript.
In 2005, Jorge Hirsch, a physicist at the University of California-San Diego, proposed the h-index to measure the productivity and impact of a scientist's research. The index became an instant success.
Despite its numerous limitations-the measure is sensitive to career stage, heavily discounts "blockbuster" articles, and can only increase with experience - the h-index enjoys considerable popularity.
"Since effort cannot in general be monitored, reward cannot be based upon it. So a scientist is rewarded not for effort, but for achievement."
Economics provides some insight regarding who engages in fraud and the type of fraud most likely to be caught. Models predict, for example, that fraud is more likely to be caught in the case of radical research, such as that put forward by Woo Suk Hwang and Jan Hendrik Schon, but that fraud is more common in incremental research. Economics, however, can only go so far. One is still left with the question of why a high-profile researcher would engage in fraudulent research of a radical nature that has a high probability of being scrutinized. One suspects that such behavior is irrational at its core and perpetrated by researchers who either seek to gratify their ego by making unsubstantiated claims or by researchers who are sufficiently irrational to drastically underestimate the probability of detection.
This finding is consistent with an earlier finding of Murray and her coauthor Scott Stern that knowledge that is embodied in both papers and patents - what are called patent-paper pairs - is cited less frequently once the patent has been issued.
The ability to keep certain findings and material for oneself (and one's students) is facilitated by the fact that publication is not synonymous with replicability. It is also facilitated by the fact that certain kinds of knowledge, especially knowledge that relates to techniques, can only be transferred at considerable cost. This is partly due to the fact that their tacit nature makes it difficult, if not impossible, to communicate in a written form. This "sticky" nature of tacit knowledge means that face-to-face contact is required for transmission. It is one reason, as we will see in Chapter 9, why innovations are clustered in certain geographic areas, such as Silicon Valley.
There is some mobility in science, but it is not that common.
Just such a person received considerable attention at the time the 2008 Nobel Prize was awarded in chemistry (to Osamu Shimomura, Martin Chalfie, and Roger Tsien) for the "discovery and development of the green fluorescent protein, GFP." GFP, used as a tagging tool in research, allows scientists to watch processes involved in cancer, neural development, and more.As is often the case, a fourth person, Douglas Prasher, was involved in the discovery. But in this case, the fourth person had left science and was driving a courtesy shuttle at the time the prize was awarded. He took the $8.50-an-hour job after a year of unemployment following the loss of aresearch position on a NASA-funded life science project. But it was Prasher who had cloned GFP, when hardly anyone understood its potential, and who had given it to Chalfie and Tsien when he realized that he might be leaving the field of bioluminescence.
But scientific productivity is not only characterized by extreme inequality at a point in time; it is also characterized by increasing inequality over the careers of a cohort of scientists, suggesting a casual process of state dependence, whereby current productivity - as measured by publications - relates to past success.
Chapter Three - Money
An eminent Harvard scientist said it well when asked by newly appointed Dean Henry Rosovsky the source of scientific inspiration. The reply (which "came without the slightest hesitation") was "money and flattery."
The salary (at the 60th percentile) for full professors at doctoral institutions was $120,867; that of associate professors was $84,931; and that of assistants was $72,672.
We see that computer scientists fare best among those in S&E, but engineering - especially at the rank of full professor - is not far behind. The biological and biomedical sciences pay almost the same as the physical sciences.
Star scientists may not earn the megabucks that sports stars do, but they earn considerably more than their peers of equal rank and five to six times as much as rookies.
Salaries in academe may be more equally distributed than in the larger society, but income inequality has been growing at a much greater rate in academe.
Funding levels, in turn, affect salary. For medical schools, the relationship can be direct, even for tenured faculty: no grant (from which to charge off salary), no pay (or reduced pay). To be more specific, in 35 percent of medical schools, tenure is accompanied by no financial guarantee for basic science faculty. In 52 percent, tenure is accompanied by a specific financial guarantee, but only in 13 percent is this guarantee for total institutional salary. Thus, increasing amounts of risk are being shifted from universities to faculty, at least in medical schools.
In a handful of countries, national policies have been implemented that award cash bonuses to individuals who publish in top international journals. The Chinese Academy of Sciences adopted such a policy in 2001. Rewards vary by institute, but they represent a large amount of cash compared with the standard salary of researchers. Bonuses are particularly high for publications in journals such as Science and Nature and, depending upon the institute, can be as high as 50 percent of salary.
It is common to attribute the dramatic increase in university patenting and licensing to the passage in 1980 of the Bayh-Dole Act, which gave U.S. universities intellectual-property control over inventions resulting from research funded by the federal government, and which universities extended to intellectual property developed from other sources of support. But attributing the increase exclusively to Bayh-Dole is far too simplistic. It ignores dramatic changes that occurred in molecular biology during these years, which opened up opportunities for scientists to conduct research that has the possibility not only of advancing basic understanding but also of being "use" oriented-that is, for scientists to work in what is commonly referred to as Pasteur's Quadrant.
Universities did not stand by passively during the debate that accompanied Bayh-Dole. Rather, a number of universities, including Harvard, Stanford, the University of California, and MIT, actively lobbied for passage of the act.
One of the most successful was that at Stanford, developed by Neils Reimers in 1968.
Faculty now routinely receive a portion of the net royalty income, although the "sharing" formula varies across universities. In slightly more than 60 percent of all universities, faculty get the same percentage of royalties regardless of whether the sum is $5,000 or $50 million. The average for such arrangements is 42 percent, but there is some variation. For about a third of the universities that pay out a fixed percentage, the rate is at or below 33 percent. But four out of ten universities share fifty-fifty, and a handful share more than 50 percent with faculty.
Because approximately 96 percent of university patents result in royalty payments of less than $50,000, the 49 percent is a close approximation of the average rate that faculty who patent can expect to get in these universities; for faculty working in universities with a fixed share, the average percentage, as previously noted, is 42 percent.
But the lion's share of revenue comes from medically related patents. The last year university licensing revenues were reported by field was in 1996, and in that year, among U.S. institutions reporting revenue by field, 76.7 percent of the royalty income came from patents in the life sciences.
Excluding the $650 million that NYU received for the previously mentioned sale, net royalties to universities in 2007 equaled $1,880 million.
John Hennesy, a computer scientist and the tenth president of Stanford University, realized considerable gains when MIPS Technology, a company he cofounded while on sabbatical from Stanford during the academic term 1984-1985, was acquired by Silicon Graphics for $333 million in 1992.
The pay is not that high $500-$2,500 per meeting - but it is stead, and the majority of SABs (scientific advisory boards) offer stock option to members.
The Association of University Technology Managers (AUTM) estimates that 3,376 academic start-ups were created in the United States from 1980 to 2000. Not all of these were the doing of faculty - some were the brainchildren of students.
The shaping of closer ties between the university and industry is considered to be one of the great legacies Frederick Terman ("the father of Silicon Valley") left to Stanford University. Although these ties took a variety of forms, consulting was one of the activities that Terman actively encouraged while dean of the School of Engineering and later as provost of the university.
In the words of an MIT engineer, "it is useful to talk to industry people with real problems because they often reveal interesting research questions."
Research shows that patenting and publishing go hand in hand: the number of patents a faculty member has relates to the number of articles the faculty member has published, and the number of articles published relates to the number of patents... the complementarity between patents and publications arises in part because scientists increasingly work in Pasteur's Quadrant, generating both fundamental insights and solutions to problems.
Most find that industry sponsorship comes with the price of delayed publication.
One study found that fully one-third of all articles published in fourteen leading biology and medical journals in 1992 had at least one lead author with a financial interest in a company related to the published research, but virtually none of the authors disclosed the relationship.
There is also the concern that faculty put their name on articles that have been "ghosted" for them by industry. In the case of the drug rofecoxib (Vioxx), Merck employees prepared manuscripts and subsequently recruited academics to serve as coauthors. Although 92 percent of the clinical trial articles included a disclosure of Merck's financial support, only 50 percent of the review articles contained either a disclosure of sponsorship or a disclosure indicating that the author had received financial compensation from Merck. Such unethical practices diminish the credibility of science and lower public trust in research.
Chapter Four - The Production of Research: People and Patterns of Collaboration
Slightly over half of the physicists questioned in a study of what it takes to succeed in their field chose persistence from the list of twenty-five adjectives. No other quality came close.
According to a NSF survey, scientists and engineers in academe for whom research is either the most important or second most important work activity spend 52.6 hours in a typical week working on their main job. Many scientists work even longer hours; the standard deviation was 9.1, and the maximum number of weekly hours reported was 96.
A 2006 survey of U.S. scientists found that scientists spend 42 percent of their research time filling out forms and in meetings, tasks split almost evenly between pre-grant (22 percent) and post-grant work (20 percent).
"A faculty member is only as good as his or her best postdoc"
For example, approximately 36,500 postdoctoral scientists and engineers were working in academe in graduate departments in the United States in 2008 - more than twice as many as in 1985. Almost 60 percent of the 36,500 postdocs were in the life sciences; the next most likely field for postdocs to be working in was the physical sciences.
In certain ways, the research enterprise itself at U.S. universities resembles a pyramid scheme. In order to staff their labs, faculty recruit PhD students into their graduate programs with funding and the implicit assurance of interesting research careers... the number of tenure-track positions has failed to keep pace with the large number of newly minted PhDs. It is not uncommon for recent graduates to feel that the system has not delivered what it promised.
There is clearly a need to rethink the way in which prizes are structured. Status, as the Nobel Peace Prize so aptly demonstrates, need not be conferred on one person at a time. It is time to think about creating prizes that can be shared by a team.
Chapter Five - The Production of Research: Equipment and Materials
The historian of science Derek de Solla Price writes, "If you did not know about the technological opportunities that created the new science, you would understandably think that it all happened by people putting on some sort of new thinking cap... The changes of paradigm that accompany great and revolutionary changes may sometimes be caused by inspired thought, but much more commonly they seem due to the application of technology to science"
In the United States, it is not uncommon for a scientist to have a lab with a quarter of a million dollars of equipment and materials. And this is toward the lower end; the equipment in a lab can easily exceed $1 million.
In 2008, U.S. universities spent nearly $1.9 billion on equipment out of current funds. Of this, 41 percent was spent in the life sciences, 17 percent in the physical sciences, and 23 percent in engineering.
In 2003, the average start-up package for an assistant professor in chemistry was $489,000; in biology, it was $403,071.
In terms of overall expenditures, including administrative costs, the HGP cost $3 billion. It is a commentary on the cost reduction resulting from the continued improvement of the equipment that the genome would have been sequenced at a cost of only $25 to $50 million had it been possible from the beginning of the project to use the equipment available in 2006.
One estimate put half the world's 1,400 sequencing machines in just twenty academic and research settings in 2010.
Johns Hopkins, for example, employs ninety people, including seven veterinarians, to care for their 200,000 mice
A particularly famous case of contamination was documented by Walter Nelson-Rees and colleagues, who were able to show that an extraordinarily robust cell line known as the HeLA (named after the cervical cancer donor Henrietta Lacks) had contaminated dozens of cell lines widely used in the 1970s
Approximately 180 million square feet are devoted to research in science and engineering at U.S. academic institutions. Over 45 percent of this is for research in the biological, medical, and health sciences. Engineering and the physical sciences each have claim to about 17 percent of the space, and agricultural sciences to another 16 percent. The remainder is shared by computer sciences and "other sciences."
As one scientist wrote, "I have worked in some of the best-funded (academic) laboratories in the world, and even these laboratories do not have access to fancy next-generation machines in a way that large biopharmaceutical companies do. I strongly believe that this is changing the nature of the public/private divide and the extent to which academic science manages to stay at the technological frontier."
How much loss of efficiency is there in equipment markets, and are the associated monopoly profits necessary to entice suppliers into markets where technology changes so quickly?
Fifth, how much does a scientist's success depend upon having a monopoly on new types of equipment or securing a monopoly on a time slot on a scarce resource such as a telescope or on a submergence vehicle such as Alvin?
Chapter Six - Funding for Research
Stanford University receives approximately $759 million a year in support of research... this represents 23 percent of university revenues.
The typical lab at a public university, for example, composed of eight researchers-a faculty principal investigator (PI), three postdoctoral fellows (postdocs), and four graduate students - plus an administrator has annual personnel costs of just over $400,000 after fringe benefits but before indirect costs. (That is $53,000 for each postdoc, $35,000 for each graduate student, $53,300 for the administrator, and, at 50 percent of the faculty member's salary, $55,850 for the PL) Add in 500 mice and $18,000 a year in supplies for each researcher, and lab costs come to about $550,000 a year before one has even opened the equipment catalogue, which could easily set the lab back another $50,000 to $100,000.
The rationale for public investment in research and development (R&D) is thus twofold: to provide the needed resources for basic research and to invest in research that provides for openness.
The public's rationale for supporting scientific research also rests on the importance of R&D to specific outcomes deemed socially desirable and not directly provided by the the market, such as national defense and better health.
In 2009, U.S. universities spent almost $55 billion on research. The largest contributor to research by far was the federal government (59.3 percent), followed by universities themselves (20.4 percent). Considerably less came from state and local governments (6.6 percent), industry (5.8 percent), and other sources (7.9 percent) such as private foundations.
Between the late 1950s and the early 1970s, the number of doctorate-granting institutions in the United States grew from 171 to 307.
The start button was pushed again with the passage of the American Recovery and Reinvestment Act of 2009, which provided $21 billion for science and engineering research and infrastructure support, much of which was targeted for universities. The act was revolutionary in the sense that it was the first time that funding for research had been specifically provided as a countercyclical measure. Moreover, heretofore research funding had been procyclical.
By far the most controversial of these agreements was struck between the Department of Plant and Microbial Biology at the University of California-Berkeley and Novartis in 1998. In exchange for up to $25 million in research support over a five-year period and access to Novartis's gene-sequencing technology and DNA database on plant genomics, Novartis was given first rights to negotiate licenses to patents on a proportion of the discoveries made in the department.
Some nonprofit foundations support a wide range of initiatives, research (including university research) being but one of them. Currently, the largest of these is the Bill and Melinda Gates Foundation. Its net worth, which was over $29 billion in 2006, was significantly increased that year when Warren Buffet signed a letter of intent pledging $31 billion to the foundation in Berkshire Hathaway shares.
Perhaps no other nonprofit organization has had as powerful an impact on academic research in the United States as the Howard Hughes Medical Institute (HHMI). Established in 1953 by the late aviator and highly eccentric engineer, industrialist, and movie producer Howard Hughes, the institute acquired a stronger footing when it sold the Hughes Aircraft Company to General Motors in 1985, thus establishing the institute's endowment at $5 billion. At the close of the 2010 fiscal year, the endowment was valued at close to $14.8 billion (down from $18.7 billion in fiscal 2007). By law, HHMI is required to distribute 3.5 percent of its assets each year. It has done so by supporting between 300 and 350 HHMI investigators at research universities, funding a number of training programs, and establishing the "farm" - the Janelia Farm Research Campus, in Ashburn, Virginia, which opened in 2006 with the goal of bringing twenty-five interdisciplinary teams together to study neural circuits and imaging.
The institute prides itself on "supporting people, not projects."
Moreover, foundations that fund grants out of their endowment can experience severe problems when the stock market takes a deep dive, as it did in 2001 and again in 2008.
Foundations that invested almost exclusively with Bernard Madoff, for example, found their balance sheet at close to zero in 2008. The Picower Foundation, which reported assets of almost $1 billion in 2007, announced in late 2008 that it would "cease all grant making effective immediately."
A second reason universities are picking up a larger share of the cost for research relates to start-up packages. As already discussed, in recent years it has become the norm for universities to provide start-up packages for new hires. Universities can easily spend $10 million a year on such packages.
Over the past ten to fifteen years, China has become a major force in world R&D. Indeed, by 2007 (the latest year for which good data are available), China was spending approximately $100 billion a year on R&D, or 10 percent of the world R&D total, leading it to rank third behind the United States (33 percent of world R&D) and Japan (13 percent of world R&D). China's increasing commitment to R&D can readily be seen by tracking the percentage of its gross domestic product (GDP) that it devotes to R&D. In 1998, it was 0.7 percent. By 2007, it had more than doubled to 1.49 percent. The United States, by comparison, spends 2.68, and Japan 3.44.
A third of the research funds going to universities come from industry - an impressive figure compared with that for other countries (see Table 6.1). The high percentage reflects the common practice for Chinese universities to have joint research programs with firms. This close relationship is one reason that Chinese universities perform a smaller percentage of basic research (38 percent) compared with that performed by academic institutions in the United States (56 percent)
A case in point is Tian Xu, a professor of genetics at Yale University (and a Howard Hughes Investigator) who has been coming back to Fudan University in Shanghai since 2002. What really brought him back was the opportunity to run a genetics program, the scale of which is unimaginable in the United States, and to work with young Chinese scientists. To wit: Xu has facilities to house thousands of mice (45,000 cages, to be precise) in two separate buildings - something that would be absolutely impossible for a faculty member to have in the United States, not only because of the annual cage costs ($11 million plus) but because it would simply not be possible to get that much research space at a U.S. university
An editorial in Science, signed jointly by the deans of the Schools of Life Sciences at Tsinghua University and Peking University (the two most highly rated universities in China), charges that "rampant problems in research funding - some attributable to the system and others cultural-are slowing down China's potential pace of innovation."
"To obtain major grants in China, it is an open secret that doing good research is not as important as schmoozing with powerful bureaucrats and their favorite experts."
"Defense-related funding from the federal government altered the focus of university research beginning with World War II. It also contributed to the
expansion of several universities, including the Massachusetts Institute of Technology and the California Institute of Technology. Other universities were quick to learn from their sister institutions and used postwar defense contracts to propel themselves into the all-star league. Stanford was an early example of this; more recently, the Georgia Institute of Technology and Carnegie Mellon have benefited from defense-related research."
The majority of these funds come from just four federal agencies: the National Institutes of Health (NIH), the National Science Foundation (NSF), the Department of Defense (DOD), and the Department of Energy (DOE), in that order. In addition, the U.S. Department of Agriculture (USDA) and National Aeronautics and Space Administration (NASA) provide over a $1 billion a year in support of university research.
approximately six out of ten federal dollars coming to U.S. universities in support of research are distributed through the process of peer review
Historically, the NIH review process has put considerable weight on past accomplishments, which are enumerated on a standardized NIH biosketch form. Results from the previous grant (if there was one) also play an important role in evaluation. The presence of demonstrated expertise and strong preliminary data play an especially key role in the review process: "No crystal, no grant." A major reason that universities provide start-up funds is to permit the newly hired faculty member time to continue the process of collecting preliminary data for an NIH proposal. The "lineage" of the scientist is often noted, in terms of where the scientist trained and in whose lab the scientist did his or her postdoc work. Researchers must also demonstrate that they have adequate space at their university in which to conduct the research.
Anywhere between 10 and 40 percent of the NIH applications are funded.
Money for earmarks for university research has grown in leaps and bounds ever since. In 2008, earmarks equaled $4.5 billion or 14 percent of all federal funding for research.
But most universities that receive funds hire lobbyists to make their case in Washington, D.C. Moreover, it is not only the second string who lobby. Despite the public disdain that most elite universities hold for earmarks, they too engage in the practice. In fiscal year 2003, 90 percent of AAU institutions accepted at least one earmark and received a total of $336 million in earmarks. Despite their efforts, earmarking redistributes funds away from top research universities toward lower-ranked institutions.
Congressional representation also affects NIH allocations and (indirectly) the distribution of grants. Powerful congressmen, for example, can provide guidance on the allocation and disbursement of appropriated funds, direct reallocations among various NIH institutes, and support funds for specific diseases. Having an additional member on the appropriate subcommittee of the House Appropriations Committee that deals with the NIH budget has been shown to increase NIH funding to public universities in the member's state by 8.8 percent.
Prizes have been particularly embraced by the private sector and philanthropists. A 2010 McKinsey study reported that more than sixty prizes of at least $100,000 each debuted between 2000 and 2007, representing almost $250 million in prize money.
But block grants with no strings attached have costs. There is no built-in incentive for faculty to remain productive throughout their research career when neither funding nor salary depend on performance. Moreover, the research agenda is often set by the director of the laboratory or by full professors in the university. As a result, younger faculty may be constrained from following leads they consider promising and must wait for their senior colleagues to retire prior to leading a research effort. Perhaps most important, the no-strings-attached approach fails to meet the criterion of accountability.
Getting money from a venture capitalist is not that different from getting money from a funding agency-both require making a strong pitch.
A competitive funding system can also discourage risk taking. Grants are often scored on their "doability," selected because they are "almost certain to 'work.'" To quote the Nobel laureate Roger Kornberg, "If the work that you propose to do isn't virtually certain of success, then it won't be funded."
The underlying incentive system encourages risk aversion on the part of the PI: failure is not rewarded. Future funding clearly depends on obtaining successful outcomes during the current grant period.
The rubric for today's faculty has gone from publish or perish to "funding or famine," to use a phrase coined by Stephen Quake.
The way funding is structured, at least that at NIH, also discourages scientists from taking up new research agendas during the course of their career. Because renewals have a much higher chance of receiving a thumbs up, researchers stay with a known course and specialize in a line of research over their career.
Neither has the competitive grants system proved to be friendly to the young... First, the need for preliminary results biases funding decisions towards more established researchers... Second, more than 70 percent of new investigators must resubmit their proposals before receiving funding... Third, people increasingly are older at the time that they get a faculty position.
In an effort to increase risk taking, the NIH created the Pioneer and Eureka awards.
Third, tuition for graduate students (which is included in grants) was increasing. The increase provided a way for universities to get more federal funds.
Mansfield found that 11 percent of the new products and 9 percent of new processes... could not have been developed in the absence of recent academic research. He uses this data to estimate social rates of return of the magnitude of 28 percent.
At least three factors may account for why the HHMI system appears to do better than the NIH system: it evaluates people, not projects; it funds individuals for a longer period of time than does the typical NIH grant; and it is reasonably forgiving of "failure," at least the first time the individual comes up for review.
Chapter Seven - The Market for Scientists and Engineers
When the data are further differentiated by race, we find that the decline is largely among white men. The number of Asian citizens receiving PhDs has increased slightly over time, as has the number of African Americans and Hispanics.
Why are departments reluctant to provide information concerning the placement of their graduates? A cynic would point out that the research enterprise has come to rely on the 120,000 graduate students... to staff their labs. They are cheap - and temporary. Placement data could discourage potential applicants and put faculty research in jeopardy be killing the geese that incubate the golden eggs.
Predictions of shortages of scientists and engineers occur with some frequency, despite evidence to the contrary... First, in 1989, the National Science Foundation predicted "looming shortfalls" of scientists and engineers in the next two decades... By 1992, it was abundantly clear that the shortage had failed to materialize. The House Committee on Science, Space, and Technology's Subcomittee on Investigation and Oversight conducted a formal investigation, leading to considerable embarrassment at NSF. The next director apologized to Congress, acknowledging that "there was really no basis to predict a shortage."
Third, shortages are often predicted by groups who have a vested interest in attracting more students to graduate school and into careers in science and engineering. To quote Michael Teitelbaum, "On the issue [of shortages] where one stands depends on where one sits." Most of the assertions come from four groups: universities and professional associations, government agencies, firms that hire scientists and engineers, and immigration lawyers.
However, their strategy changed in the first decade of this century... instead, the underlying theme of these reports is that the United States is losing its dominance in science and engineering... A prime example is the Rising above the Gathering Storm report, released by the National Academy of Sciences in 2006.
...there is almost unanimous agreement that there is not a shortage of postdoctoral fellows.
The postdoc position is often described as a holding tank, where individuals sit until the market improves.
The academic market is a buyer's market, and has been for a number of years - given the strong preference of many new PhDs and postdocs to take a job at a university.
Fourth, the high cost of start-up packages make universities very selective in hiring. It is better to hire one highly productive scientist than two whose combined productivity may be slightly higher but for whom the combined costs are much higher.
While the hiring of one's own PhDs is relatively rare in the United States, the practice is common in Europe.
Mobility, or the threat of mobility, plays a key role in determining salary. Indeed, one of the primary ways by which faculty receive salary increases in the United States is to court an offer from another university, and thereby receive a counter offer from their own university.
A distinguishing characteristic of the market for scientists and engineers is the presence of cohort effects... to put it succinctly, there can be a right time for getting a PhD and a not-so-right time.
Approximately one out of ten recent PhDs was either working part time, unemployed or out of the labor force.
From the point of view of the faculty member and the university, the system works.
Chapter Eight - The Foreign Born
Forty-four percent of the PhDs awarded by U.S. institutions in science and engineering are to foreign students on temporary visas... 7.5% of science and engineering PhDs working in the United States in 2003 were born in the People's Republic of China.
In 2003... approximately 15% of the S&E doctorates in tenure-track positions in the US had become citizens through the process of naturalization.
A 2007 study of 95 US universities identified 6,199 individuals from mainland China on the faculty.
There are thirty-three Israeli computer scientists in top-forty US departments for every 100 Israeli computer scientists at Israeli universities.
Almost half of the noncitizens receiving a PhD in the US come from just three countries: China, India, and South Korea.
There is also some evidence that students are attracted to institutions having faculty of the same ethnicity... a clever piece of detective work established that foreign students are also more likely to work for faculty of the same ethnicity than to work for native-born faculty.
The majority of foreign students who come to the US to earn a PhD stay... stay rates have increased over time.
There is evidence that graduate schools give preferential treatment to native applicants over foreign applicants.
Some displacement has occurred in academe, but it is fairly minimal and concentrated primarily in postdoc positions.
The number of PhD degrees that China is awarding went from virtually zero in 1985 to over 12,000 in 2003, the last year for which there is good data.
It is estimated, for example, that in 2002, there were 884,000 bachelor's degrees awarded in China in S&E compared with 475,000 in the US.
Chapter Nine - The Relationship of Science to Economic Growth
People not only learned to use science to advance technology, they learned how to use technology to advance science.
The breakthrough of the industrial revolution was that science and technology began to reinforce each other.
According to the economist Paul Romer... "For a nation the choices that determine whether income doubles with every generation, or instead with every other generation, dwarf all other policy concerns."
Nowhere is the contribution of public research more clear-cut than in the areas of pharmaceuticals. Three-quarters of the most important therapeutic drugs introduced between 1965 and 1992 had their origins in public sector research.
It also requires considerable investment and know-how to translate basic research into new products and processes. Universities and public research institutes are not organized or governed to excel in bringing new products and processes to market. Firms are.
It is also important to note that just because the research was done at a public institution it does not follow that new products and processes would not have arisen had it not been for research conducted in the public sector - or research conducted at the particular public institution.
Public research has more of an impact on large firms than on small firms, with one exception: start-ups consistently report benefiting from public research.
Collectively, industry contributed about 6.8 percent of the articles published in the US in 2008.
Close to 40 percent of all PhDs trained in science and engineering work in industry in the US.
To quote the physicist J. Robert Oppenheimer, "The best way to send information is to wrap it up in a person."
But others point out that at least part of the blame belongs with academe, noting that for "big" things to happen, scientists must quit working with their own group and begin working in interdisciplinary teams - that's where the gold lies. But the incentive system has not encouraged this. Rather, the grant culture, until recently, has encouraged scientists to specialize and create a niche.
Chapter Ten - Can We Do Better?
The system that has evolved discourages faculty from pursuing research with uncertain outcomes. Lack of success can mean that one's next grant will not be funded. Proposals that do not look like a sure bet may be hard to get funded in the first place.
The current university research system in the US also discourages research that could disprove theories. To quote an official with a disease foundation, who asked not to be identified, "The way science careers are structured, big labs get established based on a theory or a target or a mechanism, and the last thing they want to do is disprove it and give up what they're working on. That's why we have so many targets in studying this disease. We'd like people to work on moving them from a 'maybe' to a 'no,' but it's bad for careers to rule things out: that kind of study tends not to get published, so doing that doesn't advance people's careers.
The current system may be "incredibly successful" from the perspective of faculty, as a recent report described it, but at whose cost? It is the PhD students and postdocs who are bearing the cost of the system - and the US taxpayer - not the principal investigators.
"There's no honesty at all in recruiting PhDs... There's not a hint that there's a shortage of jobs."
Here, I make seven suggestions for change that I believe could lead to a more efficient allocation of resources, especially with regard to the performance of research:
- First, require universities to report placement data as part of all research grant applications. Do not merely require that they report the information - use the outcome data in scoring proposals.
- Second, place limits on the amount of faculty time that can be charged off grants, thereby dulling the incentive for universities to hire faculty on soft money
- Third, lessen the coupling between research and training.
- Fourth, try to determine once and for all the most effective way to support graduate students and rebalance funds toward means that are more effective. Many believe that fellowships and training grants produce better outcomes for students than do graduate research assistantships.
- Fifth, monitor existing science policies and develop new policies with the understanding that policies can affect the practice of science and, by extension, research outcomes. Policies that level the playing field by making resources available to new groups of researchers can lead to an increase in output and potentially an increase in the diversity of approaches
- Sixth, if collaborative research really produces better research, change the reward system. Encourage the creation of prizes to be awarded to groups of scientists. Status, as the Nobel Peace Prize so aptly demonstrates, need not be conferred on one person at a time
- Finally, convince advocacy groups and the Congress to drop the doubling rubric. Instead of asking for a doubling of research funds, set goals: for example, spend 0.5 percent of the GDP on federally supported university research.
Three observations, however, make one question whether the current balance is efficient. First, the heavy investment that the US has made in the biomedical sciences has created a lobbying behemoth composed of universities and nonprofit health advocacy groups that constantly remind Congress of the importance of funding health-related research. There is no comparably well-established lobbying group on the part of other disciplines.
Ignoring discipline, and there are major discipline differences in cost, an analysis done by the National Institute of General Medical Sciences suggests that the marginal product of allocating another dollar to an investigator is close to zero.